Taming the spike: How Jason McLellan helped turn the tide of the pandemic
The microscopic spikes on the SARS-CoV-2 virus are fundamental to its ability to infect us. Locking these shapeshifters in place would prove fundamental to developing vaccines against it.
- 16 May 2022
- 9 min read
- by Linda Geddes
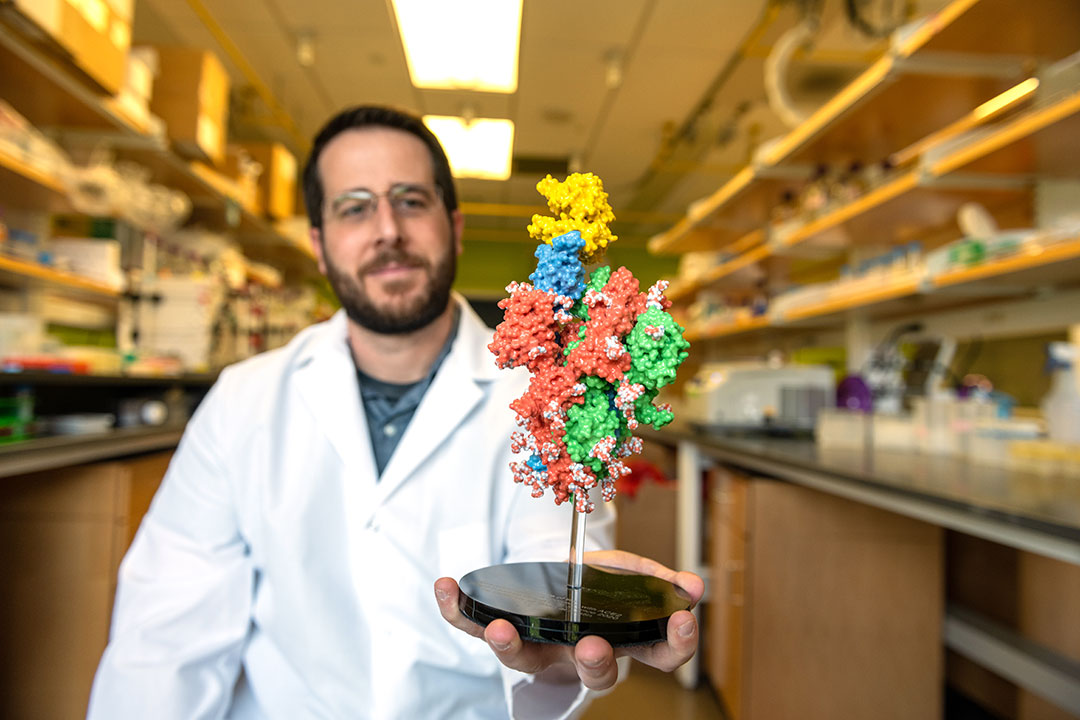
One thing everyone knows about the coronavirus is what it looks like: a sphere studded with club-shaped spikes. These jewel-like projections are what the virus uses to grab onto the cells lining our respiratory tract and infect us; they’re also what most COVID-19 vaccines are designed to mimic.
“We thought, ‘Wow, we’ve had two zoonotic coronavirus spillovers to the human population, 10 years apart; we need to prepare for the next one. Which meant we needed to figure out how to universally stabilise coronavirus spike proteins for vaccine development.”
But although they are depicted as simple blobs of protein, the reality is far cleverer and more complex: Each spike protein is the biological equivalent of a Transformer robot, undergoing dramatic conformational changes in the process of latching onto a human cell and infecting it.
Jason McLellan has devoted his career to studying the intricacies of these molecular machines, and others like them. “The structure of a protein gives the protein its function, so if we want to determine how a protein works, we need to determine its structure,” he says.
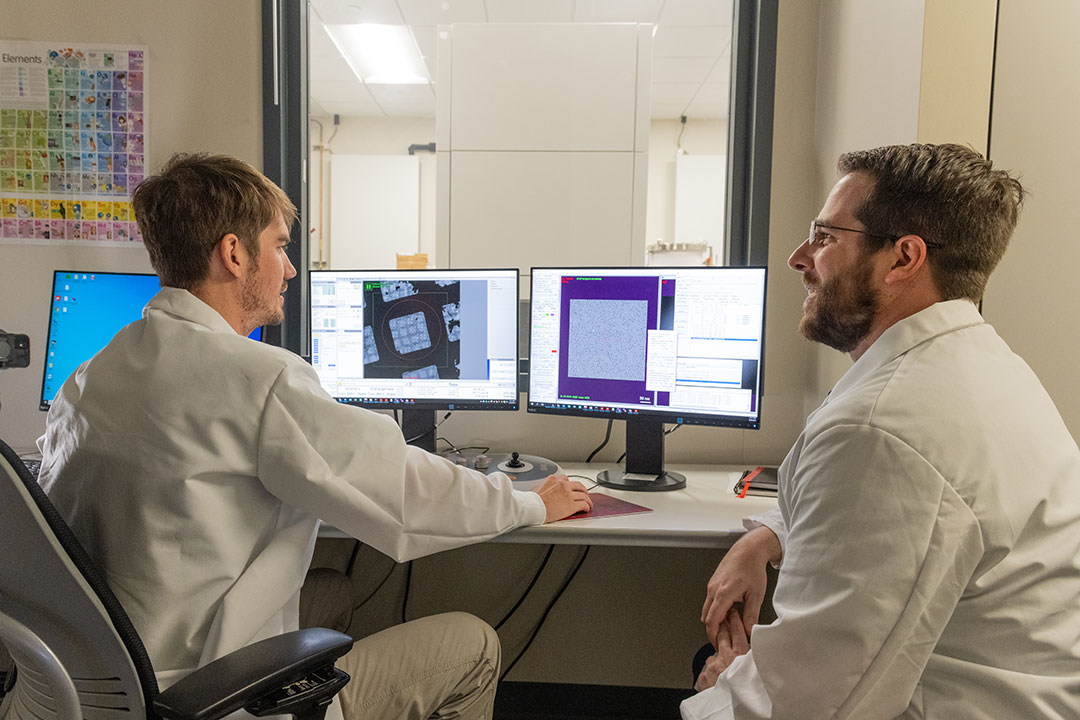
Credit: Vivian Abagiu
A professor of molecular biosciences at The University of Texas in Austin, US, his lab is unpicking the structure of viral surface proteins and investigating how to lock them in a position that optimises our immune response to them.
This has provided the foundation for many of the COVID-19 vaccines in use today – as well as several respiratory syncytial virus (RSV) vaccine candidates currently in late-stage clinical trials. Such structure-based vaccine design could also help to pre-arm us against the pandemics of the future.
McLellan had originally intended to become a doctor, but fell in love with biochemistry during his pre-medical undergraduate degree. Afterwards, he joined a lab seeking to explore the structure of proteins using a technique called X-ray crystallography.
“I was just fascinated by it, by the sense of discovery,” McLellan says. “You are potentially the first person in the history of the world to see the structure of the protein you are investigating; it’s such a thrill to see that, and to immediately begin hypothesising about its function.”
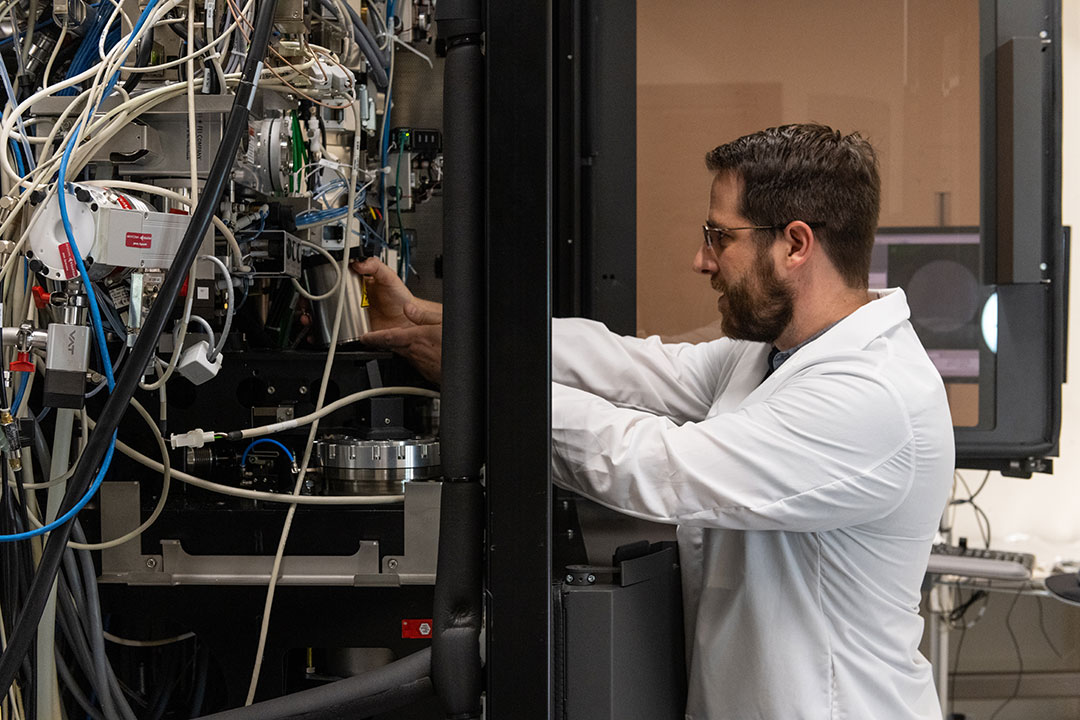
Credit: Vivian Abagiu
Proteins are the building blocks of life. Some are purely structural, whereas others function as molecular machines, catalysing the numerous reactions essential for an organism’s survival. In the case of SARS-CoV-2, its spike protein is the hand that turns the doorknob into our cells: During this process, it stretches long and thin, before partially turning itself inside out as it fuses with our cells.
These pre- and post-fusion versions of viral surface proteins have important implications for vaccine design, since pieces of them are often incorporated into vaccines. “A vaccine tries to teach our immune system to recognise a pathogen; either the whole pathogen or a piece of the pathogen. And so the better the antigen, or piece of pathogen, looks like the authentic pathogen, the better the immune response is going to be,” McLellan explains.
“If you use the post-fusion version of a protein to create a vaccine, you will raise a lot of antibodies that only bind to virus once it has fused with the host cell. They won’t prevent it from entering the cell, because the region of the protein they bind to is in a totally different conformation in the pre-fusion form.”
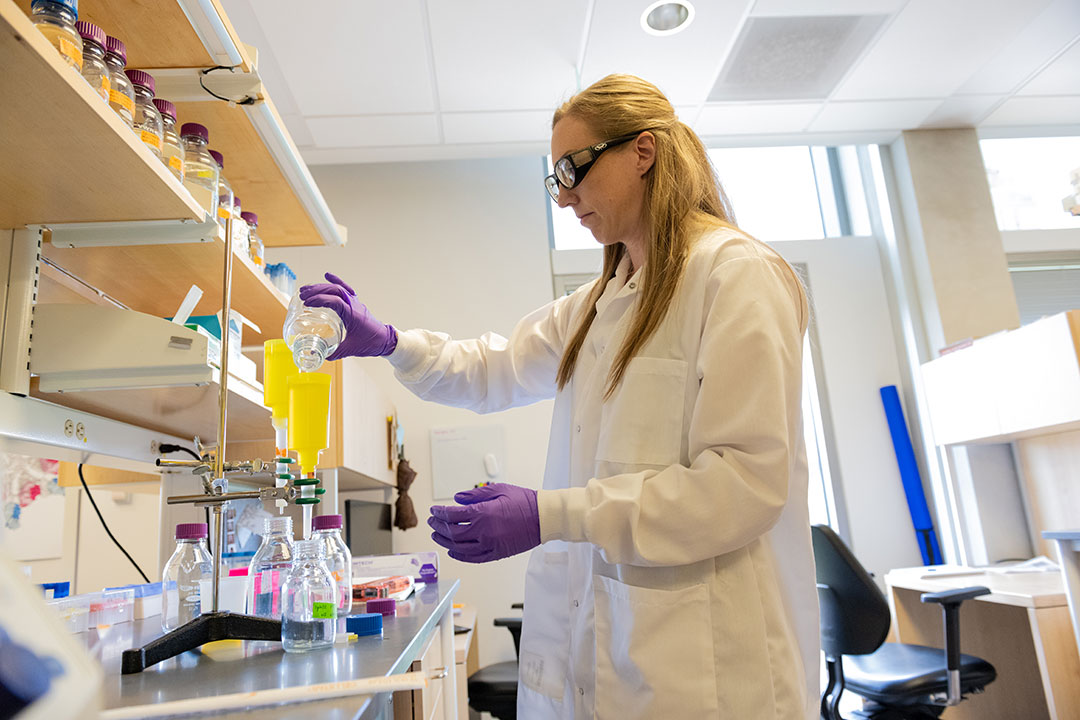
Credit: Vivian Abagiu
Ideally then, vaccine designers would present the immune system with pre-fusion forms of viral surface proteins, so that the immune system learns to recognise viruses before they start infecting cells. But first, they have to know what the different forms of these proteins look like, and engineer ways of locking them into their pre-fusion state.
After completing his PhD, McLellan joined a lab run by Peter Kwong at the National Institute of Allergy and Infectious Diseases (NIAID) Vaccine Research Center. Kwong was trying to develop a structure-based vaccine for HIV. The idea was that they would determine the crystal structure of the surface protein the HI-virus uses to fuse with our cells, and then make subtle changes to it, to make it more stable and better at stimulating an immune response.
Unfortunately, the approach didn’t work for HIV. “It wasn't clear if this was because the ideas were inherently bad, or because HIV is such a difficult virus to make a vaccine against,” McLellan said. So, the deputy director of the Vaccine Research Center, Barney Graham, suggested he try applying the approach to respiratory syncytial virus (RSV), a leading cause of infant hospitalisations and deaths, which scientists had been trying to develop a vaccine against for decades.
It turned out that one reason why some of these vaccine efforts had failed, was because the treatment used to inactivate the viral particles used in these vaccines had inadvertently locked the protein RSV uses to fuse with, and infect cells, into its post-fusion state.
Have you read?
After determining the structure of this protein, called the F protein, in both pre- and post-fusion conformations, McLellan began exploring ways of fixing it into its pre-fusion state, so that this could be used in vaccines instead.
Proteins are made up of strings of amino acids, folded into complex 3D shapes. “With these two snapshots of the pre-fusion and the post-fusion protein, I was able to engineer in changes to the amino acid sequence that would lock the protein in its prefusion shape and prevent it from adopting the post-fusion conformation,” McLellan explains.
When these stabilised proteins were incorporated into vaccines and tested in mice, they triggered a dramatic increase in antibodies capable of neutralising the virus. “I remember Barney [Graham] saying that these were the highest neutralising antibody titres he'd ever seen in his career against RSV, and we were like, ‘wow, this, this could be it’,” recalls McLellan. Further experiments in non-human primates also demonstrated a huge increase in neutralising antibody production.
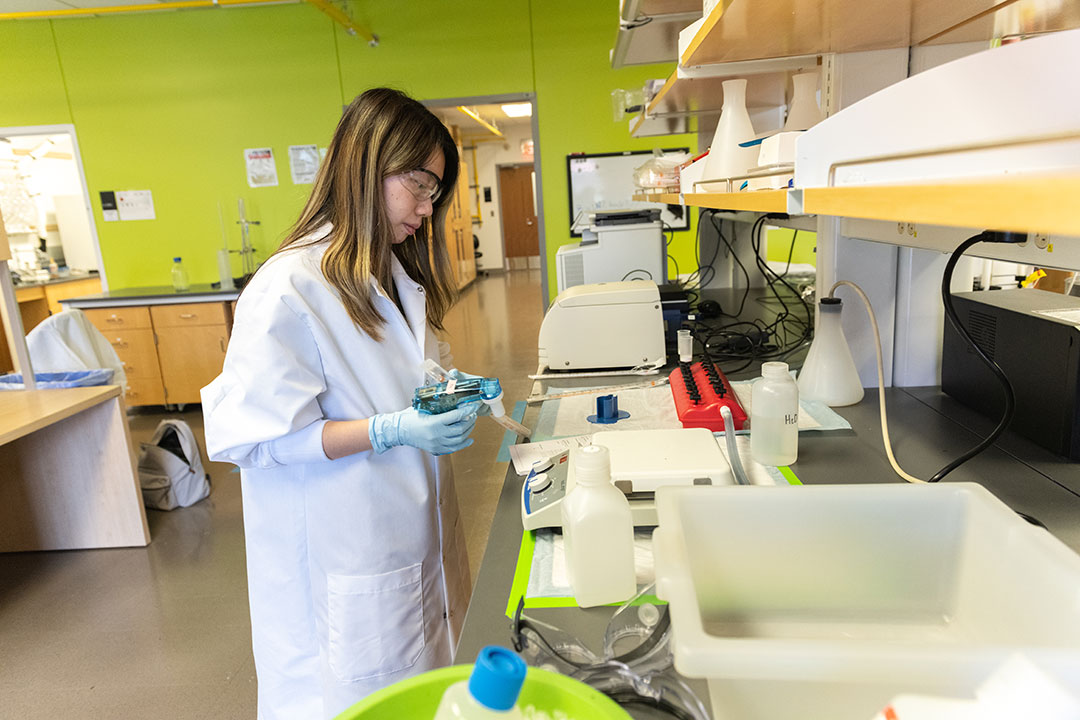
Credit: Vivian Abagiu
These results, written up and published in the prestigious journal, Science, in 2013, saw the team shortlisted for Science’s “breakthrough of the year”. It was proof-of-concept that structure-based vaccine design could work.
That same year, McLellan left the NIAID to set up his own lab at Dartmouth College in New Hampshire, but he continued to collaborate with Graham, and they started to apply everything they’d just learned about RSV to coronaviruses.
A few months earlier, Middle East Respiratory Syndrome (MERS) coronavirus had emerged in Saudi Arabia and the surrounding region. “It was really quite frightening; 35% of people infected were dying, and this came just 10 years after the emergence of SARS in 2002,” says McLellan.
“We thought, ‘Wow, we’ve had two zoonotic coronavirus spillovers to the human population, 10 years apart; we need to prepare for the next one. Which meant we needed to figure out how to universally stabilise coronavirus spike proteins for vaccine development.”
It was hard going. Unlike RSV, the MERS spike protein was difficult to work with, making even identifying the structure of the protein a struggle. “It was unstable and would fall apart, and it was difficult to produce large enough quantities to work with,” McLellan said. “It was not amenable to structural studies.”
A number of companies, including Moderna, Pfizer/BioNTech, and Novavax, used this stabilised form of the pre-fusion spike protein as the blueprint for their vaccine designs.
So instead they turned to a different coronavirus called HKU1, one of four coronaviruses that circulate in people, and one cause of the common cold. HKU1’s spike protein was better-behaved. Using a different technique called cryogenic electron microscopy, McLellan and his collaborators, including Andrew Ward at The Scripps Research Institute, managed to determine the pre-fusion structure of HKU1’s spike protein, and then used it as a blueprint to start making changes to the MERS coronavirus spike protein to stabilise and lock it into its pre-fusion state.
In the end, it only took two small changes to the amino acid sequence to achieve this. “It was incredible; just these two changes in a protein that’s about 1,200 amino acids long, gave us a boost in the amount of protein that could be produced, and caused the protein to behave and stay in the pre-fusion state,” says McLellan. They subsequently discovered that the same change could lock the spike protein of any betacoronavirus into this state.
So, when news broke of a cluster of pneumonia cases of unknown origin in Wuhan, China, at the end of 2019, McLellan and his colleagues were ready for it. Shortly after the pathogen was identified as a novel betacoronavirus, McLellan received a phone call from Graham. “He said he was going to work with Moderna to try to create the fastest mRNA vaccine ever created, and did we want to try to stabilise the SARS-CoV-2 spike protein, determine its structure, and use that to make a vaccine,” McLellan says. Immediately, he texted his laboratory team and told them to get ready.
As soon as Chinese scientists uploaded the virus’s genetic code to an open access database on 10 January 10 2020, McLellan’s team sprang into action. “Within a day, we had designed where to put the two amino acid substitutions, and within 10 days my postdoc had created the DNA to produce the protein [in a host cell],” he says. “By February 12, we had determined the structure of the stabilised SARS-CoV-2 spike protein, written the manuscript, and submitted it to Science.”
The next pandemic could be years, or even decades away, but when another killer pathogen does emerge, McLellan intends to be ready for it.
This discovery changed the course of the pandemic. Although it would take another 10 months for the first COVID-19 mRNA vaccine to be approved and delivered into a patient’s arm, a number of companies, including Moderna, Pfizer/BioNTech, and Novavax, used this stabilised form of the pre-fusion spike protein as the blueprint for their vaccine designs.
Since then, McLellan and his colleagues have produced an even more stable form of the protein, which is being used in several next-generation COVID-19 vaccines currently undergoing clinical trials, including a microneedle patch vaccine developed by a company called Vaxxas.
RSV vaccines based on their discoveries are also currently in phase 3 trials, led by GlaxoSmithKline, Pfizer and Janssen. Results from these trials are expected in the next few months.
But this may only be the beginning. Alert to the possibility of another pandemic virus emerging, McLellan and Graham are developing what they call “prototype pathogen preparedness”: They’re planning to determine the structure of key viral proteins from every virus family that could potentially cause a pandemic, and identify stabilising mutations within them – just as they did for HKU1, SARS and MERS. They’re also starting to apply the idea of structure-based vaccine design to bacterial proteins, in the hope of tackling antibiotic resistance.
The next pandemic could be years, or even decades away, but when another killer pathogen does emerge, McLellan intends to be ready for it.
More from Linda Geddes
Recommended for you
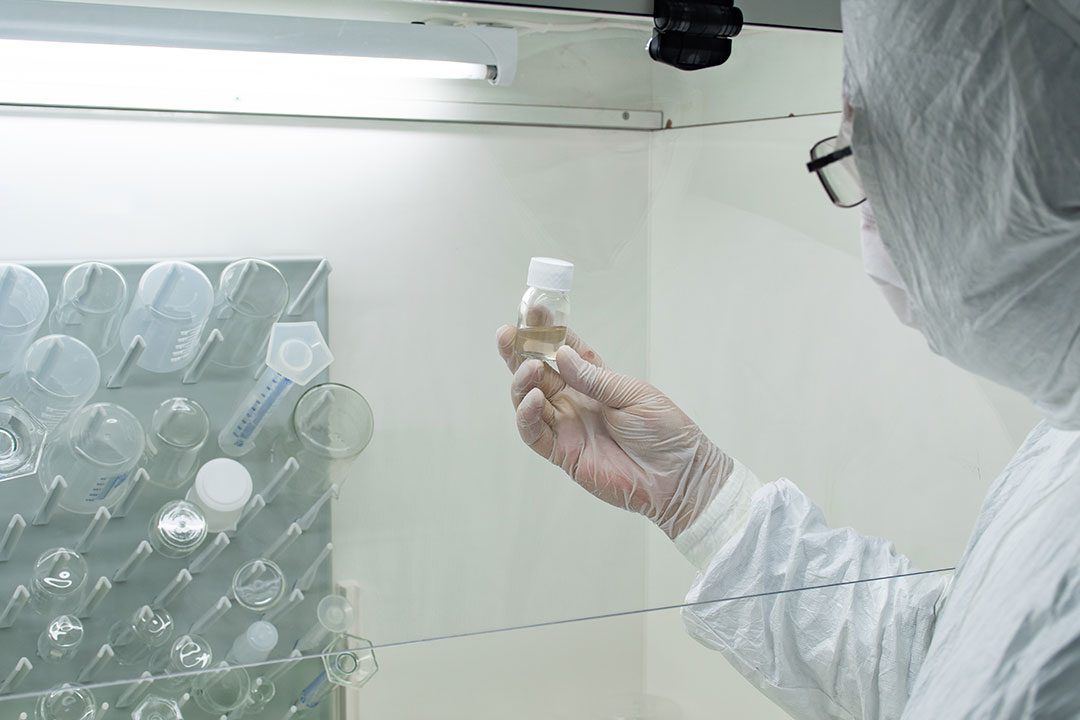
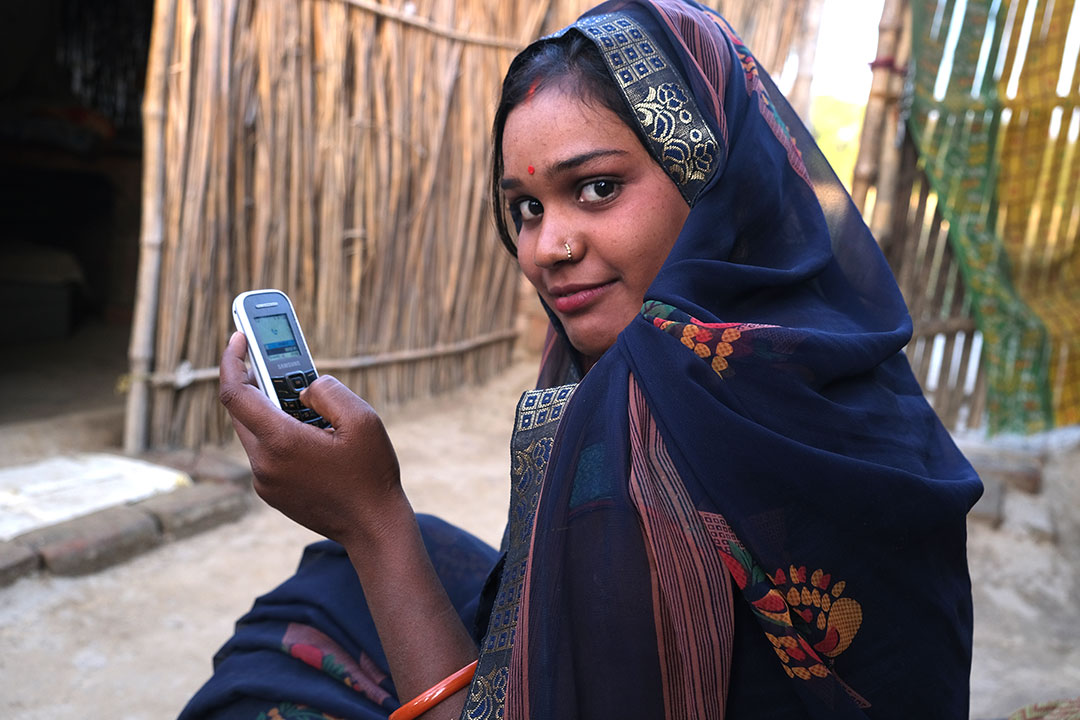
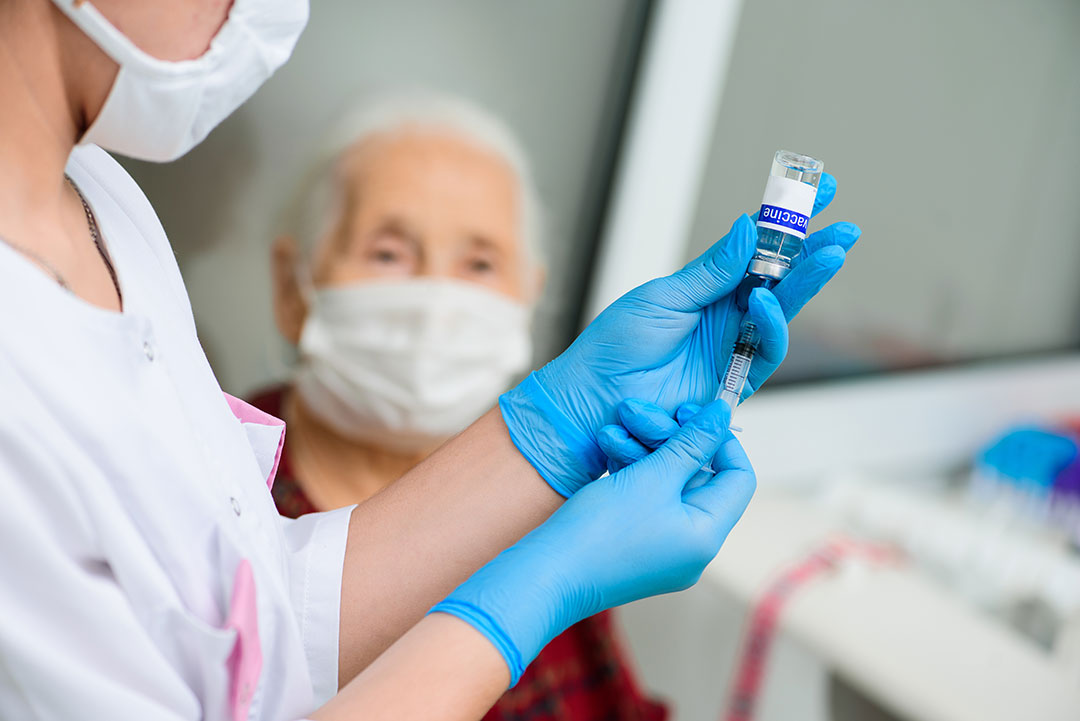